Flat-Earthers at Heart: Challenging Naive Ideas about Magnetic Fields
- Rebecca Vieyra, Chrystian Vieyra
- Jul 4, 2019
- 6 min read
Now that we've made the technological advancements in our Cyberlearning grant, it is time for us to turn to the use of our app as a learning tool. In particular, we are excited to learn about the affordances of visualizing fields in three dimensions. Some of the questions we want to answer are things like "What kinds of difficulties do learners have with understanding what a field is and what it looks like?," "What concepts do learners know/not know about magnetic fields in particular?," and "Does visualizing vectors in 3D help learners improve their visuospatial skills?"
While our testing of the app is underway with teachers and secondary through graduate students, we've found that our own conceptualizations of the world are challenged already by what we see through the camera screen.
Which way is North?
You might have learned back in middle school that the Earth can be modeled as a giant magnet. While not fully understood, the general theory as to the cause of these fields is that the Earth has a solid core that is surrounded by liquid metal. The fluid out core has convective currents within it caused by differences in temperature, and these flows are influenced in such a way by Coriolis forces (due to the spin of the Earth on its axis) that results in a more or less organized flow structure. As these charged flows move, a magnetic field is formed. The poles of this magnetic field are located near "top" and "bottom" of our planet, almost at the geographic poles upon which our planet spins, but not quite.
This model has some limitations—namely, the the geographic pole does't quite line up with the magnetic poles, and the magnetic poles aren't directly opposite each other, so the magnet we visualize at the center of the Earth is "bent." While the strength of the Earth's magnetic field is greater near the poles, which is apparent from the red coloring in the image below from the British Geological Survey, the magnetic field is far from uniform.
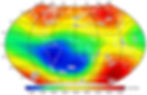
Because the magnetic poles aren't quite at the geographic poles, a compass needle might point slightly East or West than expected. This offset between the direction of magnetic North and geographic North is known as declination, and is more extreme the further north or south you go.
Further, the bedrock at each location on Earth may or may not have iron or other ferromagnetic materials beneath it, so there are places on Earth where the background field has lots of perturbations in terms of intensity and direction. However, generally speaking, most of the world can use a properly working compass to get a general sense of North.
Exploring the Earth's magnetic field is a beautiful opportunity for teaching students about Developing and Using Models, a science and engineering practice detailed in the Next Generation Science Standards that places heavy emphasis on "evaluating merits and limitations" of models and "using a model (including mathematical and computational" to generate data to support explanations, predict phenomena, analyze systems, and/or solve problems."
Conceptualizing Magnetic Fields
Typically, learners stop at the middle school model of the Earth as a bar magnet. This conceptual model is rarely challenged or enhanced, as the majority of U.S. students do not take dedicated Earth and space science courses in high school. Of those students who do take Earth and space sciences, many are not college-bound, and the courses are considered remedial. Students are often left with a vague idea that compasses can be helpful survival tools for navigating out of dark forests, but little more.
Our project is meant to advance both technology as well as learning. As such, one of our first tasks is to catalogue learners' conceptual difficulties with fields and magnets. While this area of research is not totally new (Li & Singh, 2019, Ding, Chabay, Sherwood, & Beichner, 2006; Maloney, O'Kuma, Hieggelke, & Heuvelen, 2001), most assessments of student learning have occurred at the college level and focus on the interaction between electricity and magnetism, such as the Brief Electricity and Magnetism Assessment, the Conceptual Survey of Electricity and Magnetism, or the Magnetism Conceptual Survey, which have been used widely by physics education researchers.
For our project, we are more interested in everyday conceptualizations about fields around permanent magnets. We know that these particular conceptualizations about visualized fields are likely a combination of understanding magnets and visuospatial skills (in particular, we know that geometric perspective and proportionality is important for astronomy education). Thus far, two major naive ideas have been revealed through our casual observation of users interacting with the app.
Naive Idea #1: There is no background magnetic field (or it is insignificant compared to a moderate-strength magnet).
We have observed that app users are inclined to attempt to visualize a permanent magnet's field at a distance that is too far away. Users struggle to conceptualize relative field strength of moderate-strength magnets with respect to the Earth's magnetic field, and do not know at which point the permanent magnet will overtake the visualization of the background field. We anticipate that this might be because users do not know how strong the Earth's background field is. (It is usually around 40-60 microTesla in the most heavily populated regions of the Earth). Further, even experienced physicists might not work under the idea that the inverse-cubed relationship between distance from a magnetic dipole and its field intensity means that moderate-strength permanent magnet, such as a ceramic refrigerator magnet, will drop off in field strength that will dissipate into the background field at around 5-10 centimeters.
Naive Idea #2: The background field points horizontally.
We have observed (and have experienced ourselves) a sense of surprise when users of the app realize that the Earth's background magnetic field points "down" far more than it points "North." When asking which way is geographic North, and which way is the magnetic pole in the northern hemisphere, users hold out their hands horizontally at arms-length, sometimes slightly anti-parallel to account for the declination. However, users almost never anticipate that the Earth's magnetic field is inclined.
This finding might be surprising because this incline is not minimal in the least. In Washington, DC, this incline is about 65 degrees below the horizontal!
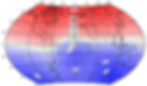
This inclination of the Earth is a product of at least two factors: (1) the magnetic field is curved around a straight dipole such as a bar magnet, and (2) the Earth's surface is rounded.
"We are all flat-Earthers at heart."
~ Colleen Megowan Romanowicz, project PI
To compare these angles, take a look at the two images below. The top one is from Washington, DC, USA, and the bottom one is from Montevideo, Uruguay (thanks to Arturo Martí).

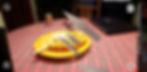
Assessing Conceptualizations
To assess these understandings, we are beginning by asking users to use the app and do some very loose think-aloud protocols. Ultimately, however, we'd like to create a concept inventory that pairs well with the kinds of things that can be learned from the app. Some of the scenarios we envision include asking learners to sketch field lines at various points on the Earth, around a bar magnet, and around a current-carrying wire from different perspectives and justify their magnitude and direction.
All of this aside, we do have some pedagogical concerns about the way we are asking learners to visualize the field:
Multiple world views: Users are confused by the x-, y-, and z-field readings on the app because they do not know if it relates to the axes of the smartphone's physical framework, the world axes of the environment, or some other localized world view. We will soon release an update that permits users to add or remove data read-outs.
Field line density versus vector visualizations: Typically, field vectors are interpreted by looking at their angle (direction) and length (magnitude). However, fields overall are also interpreted based upon the density of field lines. Because the user is responsible for visualizing each individual field vector, and may not follow any single given field line, this real sense of density is lost, or density can be artificially and erroneously created by creating multiple vectors in an area with low intensity.
Vector accuracy versus utility: Currently, the vector lines are scaled to mostly fit on the screen, but their length relative to one another is proportional to the actual total magnetic field at the point and to each other. However, we are finding that there is a loss of visualization power when vectors are so long that they extend beyond the field of view or overlap in unusual ways. We will work to determine what is better for understanding changing magnitudes while not losing the authenticity of the data visualization.
In sum, we are excited that the app has the potential to surprise some of the users about their own thinking. A final note is that we are excited to see this work, which began originally firmly in the realm of physics, extended so clearly into an integrated view of geophysics. If you have any interest in helping us to test the app (on your or your students!) please reach out to us at support@vieyrasoftware.net.
This work is funded by NSF Grant #1822728. Any opinions, findings, and conclusions or recommendations expressed in this material are those of the author(s) and do not necessarily reflect the views of the National Science Foundation.
